How does the mammalian cardiovascular system meet the body’s continuous (although variable) demand for O2?
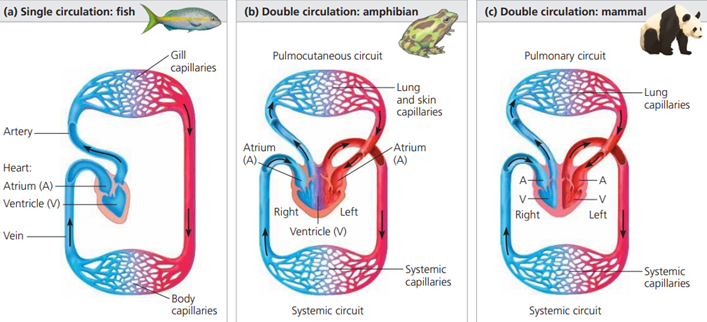
Content:
The need for specialised transport systems in animals
Types of circulatory systems
- Open circulatory systems
- Closed circulatory systems
- Single closed circulatory systems
- Double closed circulatory systems
The structure of the mammalian heart
- Why the muscular wall of the left side of the heart is much thicker than that of the right.
- A hole in heart
The cardiac cycle and the heartbeat
- Diastole
- Systole
Heart sounds
The basic rhythm of the heart
Electrocardiograms
- Heart rhythm abnormalities that commonly show up on ECGs
- Tachycardia
- Bradycardia
- Ectopic heartbeat
- Atrial fibrillation
The blood pressure
The need for specialised transport systems in animals
Multicellular organisms need transport systems to supply oxygen and nutrients to the sites where they are needed and to remove waste products from the individual cells.
In single-celled organisms, processes such as diffusion, osmosis, active transport, endocytosis and exocytosis can supply everything the cell needs to import or export.
These processes are also important in multicellular organisms, transporting substances within and between individual cells.
However, as organisms get bigger, the distances between the cells and the outside of the body get greater.
Diffusion would transport substances into and out of the inner core of the body, but it would be so slow that the organism would not survive.
Specialised transport systems are needed because:
- the metabolic demands of most multicellular animals are high (they need lots of oxygen and food, they produce lots of waste products) so diffusion over the long distances is not enough to supply the quantities needed
- the surface area to volume (SA: V) ratio gets smaller as multicellular organisms get bigger so not only do the diffusion distances get bigger but the amount of surface area available to absorb or remove substances becomes relatively smaller
- molecules such as hormones or enzymes may be made in one place but needed in another
- food will be digested in one organ system, but needs to be transported to every’ cell for use in respiration and other aspects of cell metabolism
- waste products of metabolism need to be removed from the cells and transported to excretory organs.
Types of circulatory systems
Most large, multicellular animals have specialised circulatory systems (transport systems) which carry gases such as oxygen and carbon dioxide, nutrients, waste products and hormones around the body. Most circulatory systems have features in common:
- They have a liquid transport medium that circulates around the system (blood).
- They have vessels that carry the transport medium.
- They have a pumping mechanism to move the fluid around the system.
When substances are transported in a mass of fluid with a mechanism for moving the fluid around the body it is known as a mass transport system.
Large, multicellular animals usually have either;
- an open circulatory system
- or a closed circulatory system.
Open circulatory systems
In an open circulatory system, there are very few vessels to contain the transport medium.
It is pumped straight from the heart into the body cavity of the animal.
This open body cavity is called the haemocoel.
In the haemocoel, the transport medium is under low pressure.
It comes into direct contact with the tissues and the cells.
This is where exchange takes place between the transport medium and the cells.
The transport medium returns to the heart through an open-ended vessel.
These open-ended circulatory systems are found mainly in invertebrate animals, including most insects and some molluscs.
Remember that in insects, gas exchange takes place in the tracheal system.
Insect blood is called haemolymph.
It doesn’t carry oxygen or carbon dioxide.
It transports food and nitrogenous waste products and the cells involved in defence against disease.
The body cavity is split by a membrane and the heart extends along the length of the thorax and the abdomen of the insect.
The haemolymph circulates but steep diffusion gradients cannot be maintained for efficient diffusion.
The amount of haemolymph flowing to a particular tissue cannot be varied to meet changing demands.
Closed circulatory systems
In a closed circulatory system, the blood is enclosed in blood vessels and does not come directly into contact with the cells of the body.
The heart pumps the blood around the body under pressure and relatively quickly, and the blood returns directly to the heart.
Substances leave and enter the blood by diffusion through the walls of the blood vessels.
The amount of blood flowing to a particular tissue can be adjusted by widening or narrowing blood vessels.
Most closed circulatory systems contain a blood pigment that carries the respiratory gases.
Closed circulatory systems are found in many different animal phyla, including echinoderms (animals such as sea urchins and starfish), cephalopod molluscs including the octopods and squid, annelid worms including the common earthworm, and all of the vertebrate groups, including the mammals.
Single closed circulatory systems
Single closed circulatory systems are found in a number of groups including fish and annelid worms.
In single circulatory systems the blood flows through the heart and is pumped out to travel all around the body before returning to the heart.
In other words, the blood travels only once through the heart for each complete circulation of the body.
For a single closed circulation, the blood passes through two sets of capillaries (microscopic blood vessels) before it returns to the heart.
In the first, it exchanges oxygen and carbon dioxide.
In the second set of capillaries, in the different organ systems, substances are exchanged between the blood and the cells.
As a result of passing through these two sets of very narrow vessels, the blood pressure in the system drops considerably so the blood returns to the heart quite slowly.
This limits the efficiency of the exchange processes so the activity levels of animals with single closed circulations lends to be relatively low.
Fish are something of an exception.
They have a relatively efficient single circulatory system, which means they can be very active.
They have a counter current gaseous exchange mechanism in their gills that allows them to take a lot of oxygen from the water.
Their body weight is supported by the water in which they live and they do not maintain their own body temperature.
This greatly reduces the metabolic demands on their bodies and, combined with their efficient gaseous exchange, explains how fish can be so active with a single closed circulatory system.
Double closed circulatory systems
Birds and most mammals are very active land animals that maintain their own body temperature.
This way of life is made possible in part by their double closed circulatory system.
This is the most efficient system for transporting substances around the body.
It involves two separate circulations:
- Blood is pumped from the heart io the lungs to pick up oxygen and unload carbon dioxide, and then returns to the heart.
- Blood flows through the heart and is pumped out to travel all around the body before returning to the heart again.
So, in a double circulatory system, the blood travels twice through the heart for each circuit of the body.
Each circuit to the lungs and to the body only passes through one capillary network, which means a relatively high pressure and fast flow of blood can be maintained.
How does the mammalian cardiovascular system meet the body’s continuous (although variable) demand for O2?
To answer this question, we must consider how the parts of the system are arranged and how each of these parts functions.
Let’s first examine the overall organization of the mammalian cardiovascular system, beginning with the pulmonary circuit.
Contraction of the right ventricle pumps blood to the lungs through the pulmonary arteries.
As the blood flows through capillary beds in the left and right lungs, it loads O2 and unloads CO2.
Oxygen-rich blood returns from the lungs through the pulmonary veins to the left atrium of the heart.
Next, the oxygen-rich blood flows into the heart’s left ventricle, which pumps the oxygen-rich blood out to body tissues through the systemic circuit.
Blood leaves the left ventricle through the aorta, which conveys blood to arteries leading throughout the body.
The first branches leading from the aorta are the coronary arteries (not shown), which supply blood to the heart muscle itself.
Branches further along the aorta lead to capillary beds in the head and arms (fore- limbs).
The aorta then descends into the abdomen, supplying oxygen-rich blood to arteries leading to capillary beds in the abdominal organs and legs (hind limbs).
Within the capillary beds, there is a net diffusion of O2 from the blood to the tissues and of CO2 (produced by cellular respiration) into the blood.
Capillaries rejoin, forming venules, which convey blood to veins.
Oxygen-poor blood from the head, neck, and forelimbs is channelled into a large vein, the superior vena cava.
Another large vein, the inferior vena cava, drains blood from the trunk and hind limbs.
The two venae cavae empty their blood into the right atrium, from which the oxygen-poor blood flows into the right ventricle.
The structure of the mammalian heart
Deoxygenated blood enters the right atrium of the heart from the upper body and head in the superior vena cava, and from the lower body in the inferior vena cava, at relatively low pressure.
The atria have thin muscular walls.
As the blood flows in, slight pressure builds up until the atrioventricular valve (the tricuspid valve) opens to let blood pass into the right ventricle.
When both the atrium and ventricle are filled with blood the atrium contracts, forcing all the blood into the right ventricle and stretching the ventricle walls.
As the right ventricle starts to contract, the tricuspid valve closes, preventing any backflow of blood to the atrium.
The tendinous cords make sure the valves are not turned inside out by the pressures exerted when the ventricle contracts.
The right ventricle contracts fully and pumps deoxygenated blood through the semilunar valves into the pulmonary artery, which transports it to the capillary beds of the lungs.
The semilunar valves prevent the backflow of blood into the heart.
At the same time oxygenated blood from the lungs enters the left atrium from the pulmonary vein.
As pressure in the atrium builds the bicuspid valve opens between the left atrium and the left ventricle so the ventricle also fills with oxygenated blood.
When both the atrium and ventricle are full the atrium contracts, forcing all the oxygenated blood into the left ventricle.
The left ventricle then contracts and pumps oxygenated blood through semilunar valves into the aorta and around the body.
As the ventricle contracts the tricuspid valve closes, preventing any backflow of blood.
Why the muscular wall of the left side of the heart is much thicker than that of the right.
The lungs are relatively close to the heart, and the lungs are also much smaller than the rest of the body so the right side of the heart has to pump the blood a relatively short distance and only has to overcome the resistance of the pulmonary circulation.
The left side has to produce sufficient force to overcome the resistance of the aorta and the arterial systems of the whole body and move the blood under pressure to all the extremities of the body.
The septum is the inner dividing wall of the heart which prevents the mixing of deoxygenated and oxygenated blood.
The right and left side of the heart fill and empty together.
A hole in heart
The development of the septum is not completed until after birth.
In the foetus the blood is oxygenated in the placenta, not in the lungs.
As a result, all the blood in the heart is very similar and so mixes freely.
In the days after birth, the gap in the septum closes to ensure that the deoxygenated and oxygenated bloods are kept completely separate.
Any gap remaining in the septum after the first few weeks of life is referred to as a ‘hole in the heart’ and it can often be heard with a stethoscope as a heart murmur.
Many people have a small hole in their septum without knowing about it.
However, if the hole is large it can lead to severe health problems unless it is diagnosed and repaired by surgery.
The cardiac cycle and the heartbeat
The cardiac cycle describes the events in a single heartbeat, which lasts about 0.8 seconds in a healthy human adult.
Diastole
- In diastole the heart relaxes.
- The atria and then the ventricles fill with blood.
- The volume and pressure of the blood in the heart build as the heart is filled, but the pressure in the arteries is at a minimum.
Systole
- In systole the atria contract (atrial systole), closely followed by the ventricles (ventricular systole).
- The pressure inside the heart increases dramatically and blood is forced out of the right side of the heart to the lungs and from the left side to the main body circulation.
- The volume and pressure of the blood in the heart are low at the end of systole, and the blood pressure in the arteries is at a maximum.
Heart sounds
- The sounds of the heartbeat, which can be heard through a stethoscope, are made by blood pressure and closing of the heart valves.
- The two sounds of a heartbeat are described as ‘lub-dub’.
- The first sound comes as the blood is forced against the atrio-ventricular valves as the ventricles contract,
- And the second sound comes as a backflow of blood closes the semilunar valves in the aorta and pulmonary artery as the ventricles relax.
Note:
The volume of blood each ventricle pumps per minute is the cardiac output.
Two factors determine cardiac output:
- the rate of contraction, or heart rate (number of beats per minute),
- and the stroke volume, the amount of blood pumped by a ventricle in a single contraction.
The basic rhythm of the heart
Cardiac muscle is myogenic, it has its own intrinsic rhythm at around 60 beats per minute (bpm).
This prevents the body wasting resources maintaining the basic heart rate.
The average resting heart rate of an adult is higher, at around 70bpm.
This is because other factors including exercise, excitement, and stress also affect our heart rate.
The basic rhythm of the heart is maintained by a wave of electrical excitation, rather like a nerve impulse.
- A wave of electrical excitation begins in the pacemaker area called the Sino-atrial node (SAN), causing the atria to contract and so initiating the heartbeat. A layer of non-conducting tissue prevents the excitation passing directly to the ventricles.
- The electrical activity from the SAN is picked up by the atrio ventricular node (AVN). The AVN imposes a slight delay before stimulating the bundle of His, a bundle of conducting tissue made up of fibres (Purkyne fibres), which penetrate through the septum between the ventricles.
- The bundle of is splits into two branches and conducts the wave of excitation to the apex (bottom) of the heart.
- At the apex the Purkyne fibres spread out through the walls of the ventricles on both sides. The spread of excitation triggers the contraction of the ventricles, starting at the apex. Contraction starting at the apex allows more efficient emptying of the ventricles.
The way in which the wave of excitation spreads through the heart from the SAN, with AVN delay, makes sure that the atria have stopped contracting before the ventricles start.
Electrocardiograms
You can measure the spread of electrical excitation through the heart as a way of recording what happens as it contracts.
This recording of the electrical activity of the heart is called an electrocardiogram (ECG).
An ECG doesn’t directly measure the electrical activity of your heart.
It measures tiny electrical differences in your skin, which result from the electrical activity of the heart.
To pick up these tiny changes, electrodes are stuck painlessly to clean skin to get the good contacts needed for reliable results.
The signal from each of the electrodes is fed into the machine, which produces an ECG.
ECGs are used to help diagnose heart problems.
For example, if someone is having a heart attack, recognisable changes take place in the electrical activity of their heart, which can be used to diagnose the problem and treat it correctly and fast.
Heart rhythm abnormalities that commonly show up on ECGs include:
-
Tachycardia
When the heartbeat is very7 rapid, over 100 bpm.
This is often normal, for instance when you exercise, if you have a fever, if you are frightened or angry.
If it is abnormal, it may be caused by problems in the electrical control of the heart and may need to be treated by medication or by surgery.
-
Bradycardia
The heart rate slows down to below 60bpm.
Many people have bradycardia because they are fit training makes the heart beat more slowly and efficiently.
Severe bradycardia can be serious and may need an artificial pacemaker to keep the heart beating steadily.
-
Ectopic heartbeat
Extra heartbeats that are out of the normal rhythm.
Most people have at least one a day.
They are usually normal but they can be linked to serious conditions when they are very frequent.
-
Atrial fibrillation
This is an example of an arrhythmia, which means an abnormal rhythm of the heart.
Rapid electrical impulses arc generated in the atria.
They contract very fast (fibrillate) up to 400 times a minute.
However, they don’t contract properly and only some of the impulses arc passed on to the ventricles, which contract much less often.
As a result, the heart docs not pump blood very effectively.
The blood pressure
The blood travels through the arterial system at pressures that vary as the ventricles contract.
However, the blood pressure is also affected by the diameter of the blood vessels themselves.
Narrowing the arteries is one way in which the body affects and controls local blood flow, but permanent changes can cause severe health problems.
Most people will have their blood pressure taken at some point in their lives.
Blood pressure is expressed as two figures, the first higher than the second.
But what is being measured?
Traditionally blood pressure is measured using a manual sphygmomanometer.
A cuff, which is connected to a mercury manometer (a way of measuring pressure using the height of a column of mercury), is placed around the upper arm.
The cuff is then inflated until the blood supply to the lower arm is completely cut off.
A stethoscope is positioned over the blood vessels at the elbow.
Air is slowly let out of the cuff.
The pressure at which the blood sounds first reappear as a slight tapping sound is recorded.
The first blood to get through the cuff is that under the highest pressure, in other words, when the left ventricle of the heart is contracting strongly.
The height of the mercury at this point gives the systolic blood pressure in mmHg (the height of the mercury column).
The blood sounds return to normal at the point when even the lowest pressure during is sufficient to get through the cuff.
This gives the diastolic blood pressure.
A reading of 120/80 mmHg is regarded as being normal.
More recently a simpler, digital sphygmomanometer is often used but the same principles apply.
The stethoscope is simply built into the cuff applied around the arm.