Exploring the structure and functions of the mammalian kidney
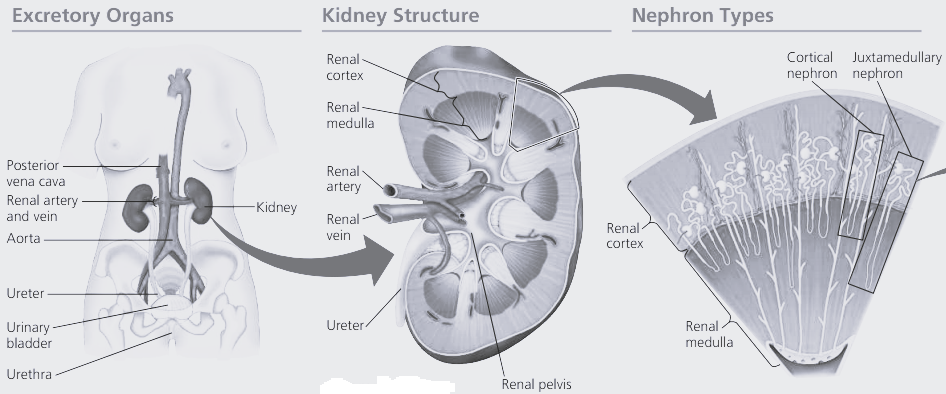
Content:
- The anatomy of the kidneys.
- Kidney structure
- Structure of the nephron
- Bowman’s capsule
- Proximal convoluted tubule
- Loop of Henle
- Distal convoluted tubule
- Collecting duct
- The functions of the nephrons
- Ultrafiltration
- Reabsorption
- The proximal convoluted tubule
- The loop of Henle
- Distal convoluted tubule
- The collecting ducts
- How long is your loop of Henle?
- The kidney and osmoregulation
- Osmoregulation
- The mechanism of ADH action
- Negative feedback control and ADH
- When water is in short supply
- An excess of water
- Water balance, and blood pressure
Human kidneys are typical of all mammalian kidneys.
They are a pair of reddish-brown organs attached to the back of the abdominal cavity.
They are usually surrounded by a thick, protective layer of fat and a layer of fibrous connective tissue.
The kidneys play two important homeostatic roles in the body;
- excretion
- and osmoregulation.
They filter nitrogenous waste products out of the blood, especially urea.
They also help to maintain the water balance and pH of the blood, and hence the tissue fluid that surrounds all the cells.
The anatomy of the kidneys.
If you put your hands on your hips, the place where your thumbs are is the approximate position of your kidneys.
The kidneys are supplied with blood at arterial pressure by the renal arteries that branch off from the abdominal aorta.
Blood that has circulated through the kidneys is removed by the renal vein that drains into the inferior vena cava.
About 90-120cm3 of blood passes through the kidneys every minute.
All of the blood in the body passes through the kidneys about once an hour.
The kidneys filter 180dm3 of blood a day, producing 1-2 dm3 of urine.
The final volume depends on many different factors.
The kidneys are made up of millions of small structures called nephrons that act as filtering units.
The sterile liquid produced by the kidney tubules is called urine.
The urine passes out of the kidneys down tubes called ureters.
It is collected in the bladder, a muscular sac that can store around 400-600cm3 of urine.
When the bladder is getting full, the sphincter at the exit to the bladder opens and the urine passes out of the body down the urethra.
Kidney structure
If you slice open a kidney, you will see three main areas;
- the cortex,
- the medulla,
- and the pelvis.
The cortex is the dark outer layer. This is where the filtering of the blood takes place and it has a very dense capillary network carrying the blood from the renal artery to the nephrons.
The medulla is lighter in colour – it contains the tubules of the nephrons that form the pyramids of the kidney and the collecting ducts.
The pelvis (which is Latin for basin) of the kidney is the central chamber where the urine collects before passing out down the ureter.
Nephrons – the functional units of the kidney
In the nephrons the blood is filtered and then the majority of the filtered material is returned to the blood, removing nitrogenous wastes and balancing the mineral ions and waler.
Each nephron is around 3 cm long and there are around 1.5 million nephrons in each kidney.
This provides the body with several kilometres of tubules for the reabsorption of water, glucose, salts, and other substances back into the blood.
Structure of the nephron
The main structures and functions of the nephron are as follows:
-
Bowman’s capsule
Cup-shaped structure that contains the glomerulus, a tangle of capillaries. More blood goes into the glomerulus than leaves it due to the ultrafiltration processes that take place.
-
Proximal convoluted tubule
It is the first, coiled region of the tubule after the Bowman’s capsule, found in the cortex of the kidney. This is where many of the substances needed by the body are reabsorbed into the blood
-
Loop of Henle
A long loop of tubule that creates a region with a very high solute concentration in the tissue fluid deep in the kidney medulla. The descending loop runs down from the cortex through the medulla to a hairpin bend at the bottom of the loop. The ascending limb travels back up through the medulla to the cortex.
-
Distal convoluted tubule
It is the second twisted tubule where the fine-tuning of the water balance of the body takes place. The permeability of the walls to water varies in response to the levels of the antidiuretic hormone (ADH) in the blood (The kidney and osmoregulation). Further regulation of the ion balance and pH of the blood also takes place in this tubule.
-
Collecting duct
The urine passes down the collecting duct through the medulla to the pelvis. More fine-tuning of the water balance lakes places the walls of this part of the tubule arc also sensitive to ADH.
The nephron has a network of capillaries around it which finally lead into a venule and then to the renal vein. The blood that leaves the kidney has greatly reduced levels of urea, but the levels of glucose and other substances such as amino acids needed by the body are almost the same as when the blood entered the kidneys (may be slightly less as some glucose will have been used for selective reabsorption). The mineral ion concentration in the blood has also been restored to ideal levels.
The functions of the nephrons
-
Ultrafiltration
The first stage in the removal of nitrogenous waste and osmoregulation of the blood is ultrafiltration.
Ultrafiltration in the kidney tubules is a specialised form of the process that results in the formation of tissue fluid in the capillary beds of the body and it is the result of the structure of the glomerulus and the cells lining the Bowman’s capsule.
The glomerulus is supplied with blood by a relatively wide afferent (incoming) arteriole from the renal artery.
The blood leaves through a narrower efferent (outward) arteriole and as a result there is considerable pressure in the capillaries of the glomerulus.
This forces the blood out through capillary wall which acts rather like a sieve.
Then the fluid passes through the basement membrane (the basement membrane is an important factor in the filtration process).
The basement membrane is made up of a network of collagen fibres and other proteins that make up a second ‘sieve’.
Most of the plasma contents can pass through the basement membrane but the blood cells and many proteins are retained in the capillary because of their size.
The wall of the Bowman’s capsule also involves special cells called podocytes that act as an additional filter.
They have extensions called pedicels that wrap around the capillaries, forming slits that make sure any cells, platelets, or large plasma proteins that have managed to get through the epithelial cells and the basement membrane do not get through into the tubule itself.
The filtrate which enters the capsule contains glucose, salt, urea, and many other substances in the same concentrations as they are in the blood plasma.
The process is so efficient that up to 20% of the waler and solutes are removed from the plasma as it passes through the glomerulus.
The volume of blood that is filtered through the kidneys in a given time is known as the glomerular filtration rate.
-
Reabsorption
Ultrafiltration removes urea, the waste product of protein breakdown, from the blood but it also removes a lot of water along with the glucose, salt, and other substances which are present in the plasma.
Many of these substances are needed by the body.
For example, glucose is used for cellular respiration and is never normally excreted.
The ultrafiltrate is also hypotonic to (less concentrated than) the blood plasma.
The main function of the nephron after the Bowman’s capsule is to return most of the filtered substances back to the blood.
-
The proximal convoluted tubule
In the proximal convoluted tubule all of the glucose, amino acids, vitamins, and hormones are moved from the filtrate back into the blood by active transport.
Around 85% of the sodium chloride and water is reabsorbed as well the sodium ions are moved by active transport while the chloride ions and water follow passively down concentration gradients. The cells lining the proximal convoluted tubule have clear adaptations:
- they are covered with microvilli, greatly increasing the surface area over which substances can be reabsorbed
- they have many mitochondria to provide the ATP needed in active transport systems.
Once the substances have been removed from the nephron, they diffuse into the extensive capillary network which surrounds the tubules down sleep concentration gradients.
These are maintained by the constant flow of blood through the capillaries.
The filtrate reaching the loop of Henle at the end of the proximal convoluted tubule is isotonic (at same concentration) with the tissue fluid surrounding the tubule and isotonic with the blood.
At this stage, over 80% of the glomerular filtrate has been reabsorbed back into the blood.
This remains the same regardless of the conditions in the body.
-
The loop of Henle
The loop of Henle is the section of the kidney tubule that enables mammals to produce urine more concentrated than their own blood.
Different areas of the loop have different permeabilities to water and this is central to the way the loop of Henle functions.
It acts as a counter current multiplier, using energy to produce concentration gradients that result in the movement of substances such as water from one area to another.
Cells use ATP to transport ions using active transport and this produces a diffusion gradient in the medulla.
The changes that take place in the descending limb of the loop of Henle depend on the high concentrations of sodium and chloride ions in the tissue fluid of the medulla that arc the result of events in the ascending limb of the loop.
-
The descending limb leads from the proximal convoluted tubule.
This is the region where water moves out of the filtrate down a concentration gradient.
The upper part is impermeable to water but the lower part of the descending limb is permeable to water and runs down into the medulla.
The concentration of sodium and chloride ions in the tissue fluid of the medulla gets higher and higher moving through from the cortex to the pyramids, as a result of the activity of the ascending limb of the loop of Henle.
The filtrate entering the descending limb of the loop of Henle is isotonic with the blood.
As it travels down the limb, water passes out of the loop into the tissue fluid by osmosis down a concentration gradient.
It then moves down a concentration gradient into the blood of the surrounding capillaries (the vasa recta).
The descending limb is not permeable to sodium and chloride ions, and no active transport takes place in the descending limb.
The fluid that reaches the hairpin bend is very concentrated and hypertonic to the blood in the capillaries.
-
The first section of the ascending limb of the loop of Henle
It is very permeable to sodium and chloride ions and they move out of the concentrated solution by diffusion down a concentration gradient.
In the second section of the ascending limb, sodium and chloride ions are actively pumped out into the medulla tissue fluid against a concentration gradient.
This produces very high sodium and chloride ion concentrations in the medulla tissue.
Importantly, the ascending limb of the loop of Henle is impermeable to water, so water cannot follow the chloride and sodium ions down a concentration gradient.
This means the fluid left in the ascending limb becomes increasingly dilute, while the tissue fluid of the medulla develops the very high concentration of ions that is essential for the kidney to produce urine that is more concentrated than the blood.
This is a key part of the counter current multiplier system.
By the time the dilute fluid reaches the top of the ascending limb it is hypotonic to the blood again, and it then enters the distal convoluted tubule and collecting duct.
-
Distal convoluted tubule
Balancing the water needs of the body takes place in the distal convoluted tubule and the collecting duct.
These are the areas where the permeability of the walls of the tubules varies with the levels of ADH.
The cells lining the distal convoluted tubule also have many mitochondria so they are adapted to carry out active transport.
If the body lacks salt, sodium ions will be actively pumped out of the distal convoluted tubule with chloride ions following down an electrochemical gradient.
Water can also leave the distal tubule, concentrating the urine, if the walls of the tubule are permeable in response to ADH.
The distal convoluted tubule also plays a role in balancing the pH of the blood.
-
The collecting ducts
The collecting duct passes down through the concentrated tissue fluid of the renal medulla.
This is the main site where the concentration and volume of the urine produced is determined.
Water moves out of the collecting duct by diffusion down a concentration gradient as it passes through the renal medulla.
As a result, the urine becomes more concentrated.
The level of sodium ions in the surrounding fluid increases through the medulla from the cortex to the pelvis.
This means water can be removed from the collecting duct all the way along its length, producing very hypertonic urine when the body needs to conserve water.
The permeability of the collecting duct to water is controlled by the level of ADH, which determines how much or little water is reabsorbed.
How long is your loop of Henle?
The ability of animals to produce very concentrated urine depends on several factors, one is the length of the loop of Henle.
As you have seen the loop of Henle develops the concentration gradient across the kidney medulla, meaning water can leave the collecting duct all the way through, concentrating the urine as it goes.
Fish have no loop of Henle and cannot produce urine that is more concentrated than their blood.
Desert animals tend to have lots of nephrons that have very long loops of Henle that travel deep into the medulla.
The kidney and osmoregulation
The mammalian kidney has a vital excretory function it removes urea, the nitrogenous waste product of metabolism from the body.
The kidney, however, also plays another important homeostatic role in the body it is the main organ of osmoregulation.
This involves controlling the water potential of the blood within very narrow boundaries, regardless of the activities of the body.
Eating a salty meal, drinking large volumes of liquid, exercising hard, running a fever, or visiting a very’ hot climate can all pull osmotic stresses on the body.
It is very important to keep the waler potential of the tissue fluid as stable as possible, because if water moves into or out of the cells by osmosis it can cause damage and even death.
Osmoregulation
Every day the body has to deal with many unpredictable events.
The water potential of the blood has to be maintained regardless of the water and solutes taken in as you eat and drink, and the water and mineral salts lost by sweating, in defaecation, and in the urine.
Changing the concentration of the urine is crucial in this dynamic equilibrium.
The amount of water lost in the urine is controlled by ADH in a negative feedback system.
ADH is produced by the hypothalamus and secreted into the posterior pituitary gland, where it is stored.
ADH increases the permeability of the distal convoluted tubule and, most importantly, the collecting duct to water.
You will be concentrating on the effect of ADH on the collecting duct walls.
The mechanism of ADH action
ADH is released from the pituitary gland and carried in the blood to the cells of the collecting duct where it has its effect.
The hormone does not cross the membrane of the tubule cells.
It binds to receptors on the cell membrane and triggers the formation of cyclic AMP (cAMP) as a second messenger inside the cell.
A second messenger is a molecule which relays signals received at cell surface receptors to molecules inside the cell.
The cAMP causes a series of events:
- Vesicles in the cells lining the collecting duct fuse with the cell surface membranes on the side of the cell in contact with the tissue fluid of the medulla.
- The membranes of these vesicles contain protein based water channels (aquaporins) and when they are inserted into the cell surface membrane, they make it permeable to water.
- This provides a route for water to move out of the tubule cells into the tissue fluid of the medulla and the blood capillaries by osmosis.
The more ADH that is released, the more water channels are inserted into the membranes of the tubule cells.
This makes it easy for more water to leave the tubules by diffusion, resulting in the formation of a small amount of very concentrated urine.
Water is returned to the capillaries, maintaining the water potential of the blood and therefore the tissue fluid of the body.
When ADH levels fall, the reverse happens.
Levels of cAMP fall, then the water channels are removed from the tubule cell membranes and enclosed in vesicles again.
The collecting duct becomes impermeable to water once more, so no water can leave.
This results in the production of large amounts of very dilute urine, and maintains the water potential of the blood and the tissue fluid.
Negative feedback control and ADH
The permeability of the collecting ducts is controlled to match the water requirements of the body very closely.
This is brought about by a complex negative feedback system that involves osmoreceptors in the hypothalamus of the brain.
These osmoreceptors are sensitive to the concentration of inorganic ions in the blood and are linked to the release of the ADH.
When water is in short supply
When water is in short supply in the body, the concentration of inorganic ions in the blood rises and the water potential of the blood and tissue fluid becomes more negative.
This is detected by the osmoreceptors in the hypothalamus.
They send nerve impulses to the posterior pituitary which in turn releases stored ADH into the blood.
The ADH is picked up by receptors in the cells of the collecting duct and increases the permeability of the tubules to water.
Water leaves the filtrate in the tubules and passes into the blood in the surrounding capillary network.
A small volume of concentrated urine is produced.
An excess of water
When large amounts of liquid are taken in, the blood becomes more dilute and its water potential becomes less negative.
Again, the change is detected by the osmoreceptors of the hypothalamus.
Nerve impulses to the posterior pituitary arc reduced or stopped and so the release of ADH by the pituitary is inhibited.
Very little reabsorption of water can take place because the walls of the collecting duct remain impermeable to water.
In this way the concentration of the blood is maintained and large amounts of dilute urine are produced.
Water balance, and blood pressure
The osmoreceptors in the hypothalamus are not the only sensory receptors that exert control over the release of ADH.
It is also stimulated or inhibited by changes in the blood pressure, detected by baroreceptors in the aortic and carotid arteries.
These baroreceptors are also involved in the control of the heart rate.
A rise in blood pressure can often be caused by a rise in blood volume.
The increase in pressure is detected by the baroreceptors and in turn they prevent the release of ADH.
This increases the volume of water lost in the urine, reducing the blood volume and so the blood pressure falls.
If the blood pressure falls it can be a signal that the blood volume has fallen.
If the baroreceptors detect a fall in blood pressure there is an increase in the release of ADH from the pituitary, so the kidneys respond to reduce water loss from the body.
Water is returned to the blood and a small amount of concentrated urine is produced.