How ATP Provides the Energy that Perform Work.
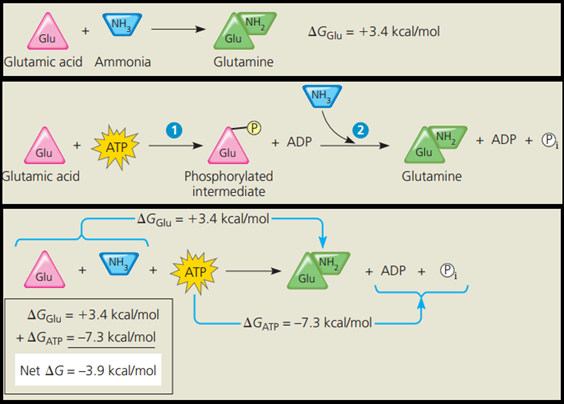
How ATP Provides the Energy that Perform Work.
The structure and hydrolysis of ATP.
ATP (adenosine triphosphate) contains the sugar ribose, with the nitrogenous base adenine and a chain of three phosphate groups (the triphosphate group) bonded to it.
In addition to its role in energy coupling, ATP is also one of the nucleoside triphosphates used to make RNA.
Also Read:
- Why the first breath taken by a new born baby is so much harder than any subsequent breaths.
- How does the mammalian cardiovascular system meet the body’s continuous (although variable) demand for O2?
- How to Detect Kidney Failure, Causes, And Effects
- Urine sample and diagnosis
The bonds between the phosphate groups of ATP can be broken by hydrolysis.
When the terminal phosphate bond is broken by the addition of a water molecule, a molecule of inorganic phosphate (HOPO32-, abbreviated as Pi throughout this site) leaves the ATP, which becomes adenosine diphosphate, or ADP.
The reaction is exergonic and releases 7.3 kcal of energy per mole of ATP hydrolyzed.
This is the free-energy change measured under standard conditions.
In the cell, conditions do not conform to standard conditions, primarily because reactant and product concentrations differ from 1 M.
For example, when ATP hydrolysis occurs under typical cellular conditions, the actual ΔG is about 13 kcal/mol, 78% greater than the energy released by ATP hydrolysis under standard conditions.
Because their hydrolysis releases energy, the phosphate bonds of ATP are sometimes referred to as high-energy phosphate bonds, but the term is misleading.
The phosphate bonds of ATP are not unusually strong, as “high-energy” may imply; rather, the reactants (ATP and water) themselves have high energy relative to the energy of the products (ADP and Pi).
The release of energy during the hydrolysis of ATP comes from the chemical change of the system to a state of lower free energy, not from the phosphate bonds themselves.
ATP is useful to the cell because the energy it releases on losing a phosphate group is somewhat greater than the energy most other molecules could deliver.
But why does this hydrolysis release so much energy?
If we reexamine the ATP molecule, we can see that all three phosphate groups are negatively charged.
These like charges are crowded together, and their mutual repulsion contributes to the instability of this region of the ATP molecule.
The triphosphate tail of ATP is the chemical equivalent of a compressed spring.
How energy is gained from ATP.
When ATP is hydrolyzed in a test tube, the release of free energy merely heats the surrounding water.
In an organism, this same generation of heat can sometimes be beneficial.
For instance, the process of shivering uses ATP hydrolysis during muscle contraction to warm the body.
In most cases in the cell, however, the generation of heat alone would be an inefficient (and potentially dangerous) use of a valuable energy resource.
Instead, the cell’s proteins harness the energy released during ATP hydrolysis in several ways to perform the three types of cellular work;
- chemical,
- transport,
- and mechanical.
For example, with the help of specific enzymes, the cell can use the high free energy of ATP to drive chemical reactions that, by themselves, are endergonic.
If the ΔG of an endergonic reaction is less than the amount of energy released by ATP hydrolysis, then the two reactions can be coupled so that, overall, the coupled reactions are exergonic.
This usually involves phosphorylation, the transfer of a phosphate group from ATP to some other molecule, such as the reactant.
The recipient molecule with the phosphate group covalently bonded to it is then called a phosphorylated intermediate.
The key to coupling exergonic and endergonic reactions is the formation of this phosphorylated intermediate, which is more reactive (less stable, with more free energy) than the original unphosphorylated molecule.
Transport and mechanical work in the cell are also nearly always powered by the hydrolysis of ATP.
In these cases, ATP hydrolysis leads to a change in a protein’s shape and often its ability to bind to another molecule.
Sometimes this occurs through a phosphorylated intermediate.
In most instances of mechanical work involving motor proteins “walking” along cytoskeletal elements, a cycle occurs in which ATP is first bound noncovalently to the motor protein.
Next, ATP is hydrolyzed, releasing ADP and Pi.
Another ATP molecule can then bind.
At each stage, the motor protein changes its shape and ability to bind to the cytoskeleton, resulting in the movement of the protein along the cytoskeletal track.
Phosphorylation and dephosphorylation promote crucial protein shape changes during many other important cellular processes as well.
How ATP is Regenerated.
An organism at work uses ATP continuously, but ATP is a renewable resource that can be regenerated by the addition of phosphate to ADP.
The free energy required to phosphorylate ADP comes from exergonic breakdown reactions (catabolism) in the cell.
This shuttling of inorganic phosphate and energy is called the ATP cycle, and it couples the cell’s energy-yielding (exergonic) processes with the energy-consuming (endergonic) ones.
The ATP cycle proceeds at an astonishing pace.
For example, a working muscle cell recycles its entire pool of ATP in less than a minute.
That turnover represents 10 million molecules of ATP consumed and regenerated per second per cell.
If ATP could not be regenerated by the phosphorylation of ADP, humans would use up nearly their body weight in ATP each day.
Because both directions of a reversible process cannot be downhill, the regeneration of ATP from ADP and Pi is necessarily endergonic:
Since ATP formation from ADP and Pi is not spontaneous, free energy must be spent to make it occur.
Catabolic (exergonic) pathways, especially cellular respiration, provide the energy for the endergonic process of making ATP.
Plants also use light energy to produce ATP.
Thus, the ATP cycle is a key player in bioenergetics, functioning as a revolving door through which energy passes during its transfer from catabolic to anabolic pathways.
Join Enlighten Knowledge WhatsApp platform.
Join Enlighten Knowledge Telegram platform.