Enlighten Knowledge Note; The Immune System (Innate Immunity/Nonspecific defenses)
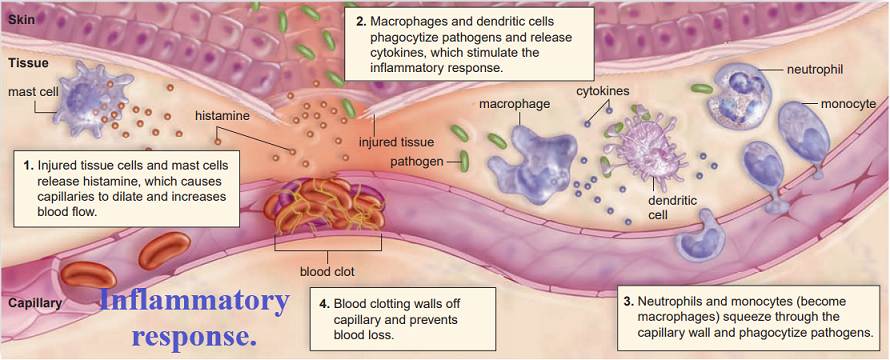
Introduction:
The body’s defense makes up the immune system, which enables an animal to avoid or limit many infections.
The first lines of defense offered by immune systems help prevent pathogens from gaining entrance to the body.
For example;
An outer covering, such as skin or a shell, blocks entry by many pathogens.
Sealing off the entire body surface is impossible, however, because gas exchange, nutrition, and reproduction require openings to the environment.
Secretions that trap or kill pathogens guard the body’s entrances and exits, while the linings of the digestive tract, airway, and other exchange surfaces provide additional barriers to infection.
To fight infections, an animal’s immune system must detect foreign particles and cells within the body.
In other words, a properly functioning immune system distinguishes nonself from the self.
How is this accomplished?
Immune cells produce receptor molecules that bind specifically to molecules from foreign cells or viruses and activate defense responses.
The specific binding of immune receptors to foreign molecules is a type of molecular recognition and is the central event in identifying nonself molecules, particles, and cells.
Two types of immune defenses are found among animals.
-
- Innate immunity
- Adaptive immunity
This concept focuses on innate immunity, the set of immune defenses common to all animals.
The remainder explores adaptive immunity and will be dealt with later.
Innate immunity/nonspecific defense.
Innate immunity is also known as the nonspecific defense.
Nonspecific defenses act rapidly to detect and respond to an infection by any and all pathogens and cancer cells.
They are;
- Barriers to entry such as the skin.
- Protective proteins such as complement and interferons.
- Phagocytes and natural killer cells.
- The inflammatory response.
The innate system involves the recognition of molecules that are conserved in particular pathogens, such as lipopolysaccharides in gram-negative bacteria.
The molecules that bind to these conserved proteins do not result from genomic rearrangements and are limited in number, but do involve recognition of invading pathogens.
The characteristic of this system is a rapid response that brings cells to the site of infection and uses soluble antimicrobial proteins to fight the pathogen.
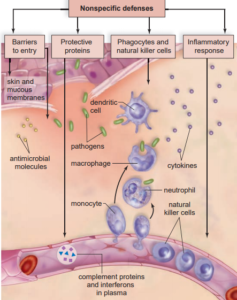
Barriers to entry such as the skin.
Barriers to entry by pathogens include non-chemical and mechanical barriers, such as the skin and the mucous membranes lining the respiratory, digestive, and urinary tracts.
The skin as a barrier to infection:
The skin is the largest organ of the body, accounting for 15% of an adult human’s total weight.
The integument not only defends the body by providing a nearly impenetrable barrier but also reinforces this defense with chemical weapons on the surface.
Oil and sweat glands give the skin’s surface a pH of 3 to 5, which is acidic enough to inhibit the growth of many pathogenic microorganisms.
Sweat also contains the enzyme lysozyme, which digests bacterial cell walls.
Epithelial cells also produce a variety of small antimicrobial peptides.
The skin is also home to many normal flora, non-pathogenic bacteria, or fungi that are well adapted to the skin conditions in different regions of the body.
Pathogenic bacteria that might attempt to colonize the skin generally are unable to compete with the normal flora.
The epidermis of the skin is approximately 10 to 30 cells thick.
The outer layer contains cells that are continuously abraded, injured, and worn by friction and stress during the body’s many activities.
Cells are shed continuously and are replaced by new cells produced in the innermost layer of the epidermis.
Mucosal epithelial surfaces also prevent the entry of pathogens
Apart from the skin, three other potential routes of entry by microorganisms and viruses must be guarded:
- the digestive tract,
- the respiratory tract, and
- the urogenital tract.
Recall that each of these tracts opens to the external environment.
Each of these tracts is lined by epithelial cells, which are continuously replaced, as are those of the skin.
A layer of mucus, secreted by specialized cells scattered between the epithelial cells, covers all these epithelial surfaces.
Pathogens are frequently trapped within this mucus layer and are eliminated by mechanisms specific to the particular tract.
Microbes are present in food, but many are killed by saliva (which contains lysozyme), by the very acidic environment of the stomach, and by digestive enzymes in the intestine.
Additionally, the gastrointestinal tract is home to a vast array of non-pathogenic normal flora, whose presence inhibits the growth of pathogenic competitors.
These non-pathogenic organisms not only outcompete pathogens, but they also may secrete substances that kill harmful agents.
Microorganisms present in inhaled air are trapped by the mucus within the smaller bronchi and bronchioles before they can reach the warm, moist lungs, which would provide ideal breeding grounds for them.
The epithelial cells lining these passages have cilia that continually sweep the mucus toward the glottis.
There the mucus can be swallowed, carrying potential invaders out of the lungs and into the digestive tract.
One of the pitfalls of smoking is that nicotine paralyzes the cilia of the respiratory system so that this natural cleaning of the air passages does not take place.
Vaginal secretions are sticky and acidic, and they also promote the growth of normal flora; all of these characteristics help prevent foreign invasion.
In both males and females, acidic urine continually washes potential pathogens from the urinary tract.
In addition to these physical and chemical barriers to pathogen invasion, the body also uses defense mechanisms such as vomiting, diarrhoea, coughing, and sneezing to expel potential pathogens.
Inflammatory Response
The inflammatory response involves several systems of the body, and it may be either localized or systemic.
An acute response is one that generally starts rapidly but lasts for only a relatively short while.
Certain infected or injured cells release chemical alarm signals most notably histamine, along with prostaglandins and bradykinin.
These chemicals promote the dilation of local blood vessels, which increases the flow of blood to the site and causes the area to become red and warm, two of the hallmark signs of inflammation.
These chemicals also increase the permeability of capillaries in the area, producing the third hallmark sign of inflammation, the edema (tissue swelling) often associated with infection.
Swelling puts pressure on nerve endings in the region, and this, in combination with the release of other mediators, leads to pain and potential loss of function, the final two hallmark signs of inflammation.
Increased capillary permeability initially promotes the migration of phagocytic neutrophils from the blood to the extracellular fluid bathing the tissues, where the neutrophils can ingest and degrade pathogens.
The pus associated with some infections is a mixture of dead or dying pathogens, tissue cells, and neutrophils.
The neutrophils also secrete signalling molecules that attract monocytes several hours later
As the monocytes differentiate into macrophages, they too engulf pathogens and the remains of the dead cells.
An inflamed area has four outward signs:
- Redness,
- Heat
- Swelling,
- Pain
The inflammatory response can be accompanied by other responses to the injury. A blood clot can form to seal a break in a blood vessel.
Antigens, chemical mediators, dendritic cells, and macrophages move through the tissue fluid and lymph to the lymph nodes.
There, B cells and T cells are activated to mount a specific defense to the infection.
Sometimes an inflammation persists, and the result is chronic inflammation that is often treated by administering anti-inflammatory agents such as aspirin, ibuprofen, or cortisone.
These medications act against the chemical mediators released by the white blood cells in the damaged area.
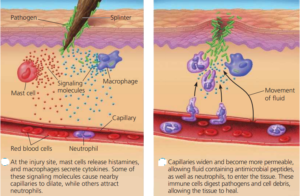
Phagocytes and Natural Killer Cells
Several types of white blood cells are phagocytic.
Neutrophils are cells that can leave the bloodstream and phagocytize (engulf) bacteria in connective tissues.
They have various other ways of killing bacteria as well.
For example;
Their granules release antimicrobial peptides called defensins.
Eosinophils are phagocytic, but they are better known for mounting an attack against animal parasites such as tapeworms that are too large to be phagocytized.
The two most powerful of the phagocytic white blood cells are
- macrophages and
- macrophage-derived dendritic cells.
They engulf pathogens, which are then destroyed by enzymes when their endocytic vesicles combine with lysosomes.
- Dendritic cells are found in the skin.
- Once they devour pathogens,
- They travel to lymph nodes
- Where they stimulate natural killer cells or lymphocytes.
- Macrophages are found in all sorts of tissues
- Where they voraciously devour pathogens
- Then stimulate lymphocytes to carry on specific immunity.
Natural killer cells are large, granular lymphocytes that kill virus-infected cells and cancer cells by cell-to-cell contact.
They do their work while specific defenses are still mobilizing, and they produce cytokines that promote specific defenses.
What makes natural killer cells attack and kill a cell?
- First, they normally congregate in the tonsils, lymph nodes, and spleen, where they are stimulated by dendritic cells before they travel forth.
- Then, natural killer cells look for a self-protein in the body’s cells.
- As may happen, if a virus-infected cell or a cancer cell has lost its self-proteins.
- The natural killer cell kills it in the same manner used by cytotoxic T cells.
- Unlike cytotoxic T cells, natural killer cells are not specific
- They have no memory.
- Their numbers do not increase after stimulation.
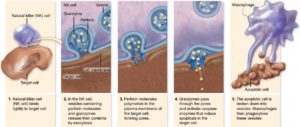
Protective Proteins
Complement is composed of several blood plasma proteins that “complement” certain immune responses, which accounts for their name.
These proteins are continually present in the blood plasma but must be activated by pathogens to exert their effects.
Complement helps destroy pathogens in three ways:
- Enhanced inflammation.
Complement proteins are involved in and amplify the inflammatory response because certain ones can bind to mast cells (a type of white blood cell in tissues) and trigger histamine release, and others can attract phagocytes to the scene.
- Some complement proteins bind to the surface of pathogens already coated with antibodies, which ensures that the pathogens will be phagocytized by a neutrophil or macrophage.
- Certain other complement proteins join to form a membrane attack complex that produces holes in the surface of some bacteria and viruses.
Fluids and salts then enter the bacterial cell or virus to the point that it bursts.
Interferons are cytokines, soluble proteins that affect the behaviour of other cells.
Interferons are made by virus-infected cells.
They bind to the receptors of noninfected cells, causing them to produce substances that interfere with viral replication.
Interferons, now available as a biotechnology product, and used to treat certain viral infections, such as hepatitis C.
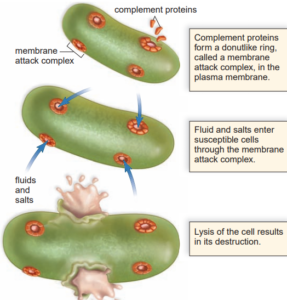
Fever
- An illness may result in a fever or an elevated body temperature.
- To treat or not to treat a fever is controversial within the medical field for, in some instances, a fever may be beneficial.
- Perhaps a fever is the body’s way of informing us that something is wrong.
- Alternatively, a fever could be part of our first line of defense.
- At times, a fever may directly participate in overcoming an illness.
For example;
- A fever can contribute to the host’s defense by providing an unfavourable environment for the invader.
Some pathogens have very strict temperature requirements, and turning up the heat on them can slow their ability to multiply and thrive.
Supporting this hypothesis is the observation that increasing the body temperature in mice has been shown to decrease death rates and shorten the recovery time linked to many infectious agents.
Other medical experts believe that the main function of a fever is to stimulate immunity.
In support of this hypothesis, a fever has been shown to limit the growth of tumor cells more severely than that of normal body cells.
This suggests either that tumor cells are directly sensitive to higher temperatures or that a fever stimulates immunity.
Heat is a part of the inflammatory response, and perhaps its main function is to jump-start the response of the body.
While data concerning the benefits of fever are inconclusive, the consensus is that an extreme fever should be treated but milder cases may be best left alone.
How innate immunity recognizes molecular patterns
Innate immunity is a response to invading pathogens that involve both soluble factors and a variety of different types of blood cells.
The innate response to invading pathogens is based on the recognition of molecules that are characteristic of the pathogen.
Collectively we call these pathogen-associated molecular patterns (PAMPs), or microbe-associated molecular patterns (MAMPs).
Examples include;
- The lipopolysaccharide (LPS) found in gram-negative bacterial cell walls
- Peptidoglycan, which is found in all bacterial cell walls
- Viral DNA and RNA.
These PAMPs are recognized by pattern recognition receptors (PRRs) that can be either soluble or on the surface of blood cells.
Cytoplasmic receptors
- Two newly characterized kinds of receptors are not membrane proteins, rather they are found in the cytoplasm.
- These are the nucleotide oligerization domain (NOD)-like receptors (NLRs) and Rig helicase-like receptors (RLRs).
- These internal receptors can recognize PAMPs in the cytoplasm of cells after phagocytosis.
- The RLRs also help in responding to viral RNA.
Soluble receptors
- Some circulating molecules can respond to molecules derived from pathogens.
- These include some of the lectin family, such as the mannose-binding lectin (MBL) protein.
- This protein is found in serum and can bind to mannose-containing carbohydrates on microbial surfaces.
- MBL is important in activating the complement system.
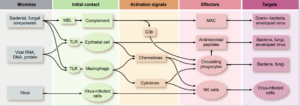