Adaptive Immunity/Specific Defenses/Acquired Immunity.
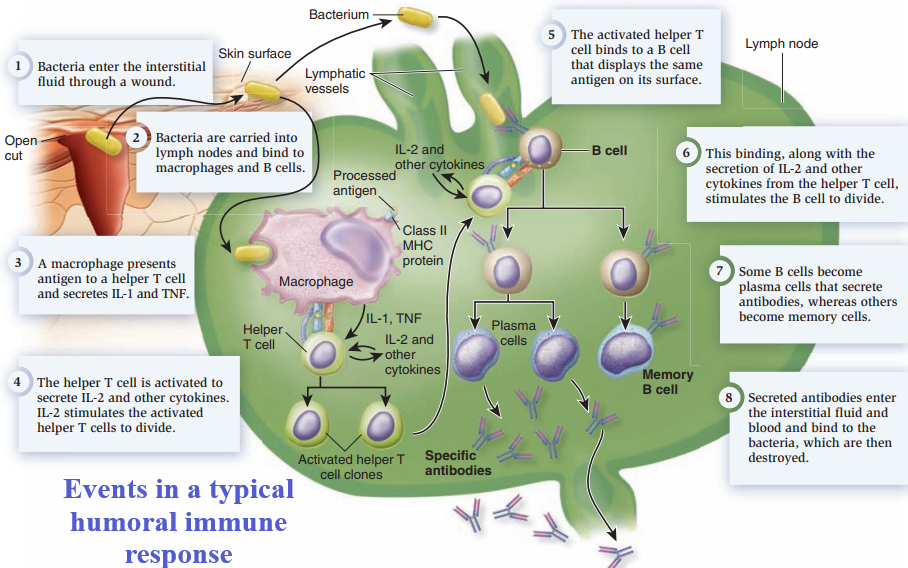
Adaptive Immunity/Specific Defenses/Acquired Immunity.
Adaptive immunity
Adaptive immunity is a vertebrate-specific defense mechanism that recognizes specific molecules as being foreign and clears those molecules from the body.
The foreign molecules recognized may be free, as in the case of toxins, or they may be on the surface of viruses or cells such as pathogenic bacteria, cancer cells, pollen, or cells of transplanted tissues and organs.
Adaptive immunity develops only after the body is exposed to the foreign molecules and, hence, takes several days to become effective.
This would be a significant problem in the case of invading pathogens were it not for the innate immune system, which combats the invaders in a nonspecific way within minutes after they enter the body.
Adaptive immunity retains a memory of the foreign molecule that triggered the response, enabling a rapid, more powerful response if that pathogen is encountered again, whereas innate immunity retains no memory of exposure to the pathogen.
Haematopoiesis gives rise to the cells of the immune system
- All the cells that are found in the blood are derived from the division, and differentiation of hematopoietic stem cells, a process called haematopoiesis.
- Embryologically, these stem cells are initially found in the yolk sac, then migrate to the fetal liver and spleen, and finally to the bone marrow.
- Stem cells give rise to lymphoid progenitors and myeloid progenitors.
- A lymphoid progenitor, in turn, gives rise to both the B and T lymphocytes as well as to natural killer cells.
- A myeloid progenitor gives rise to all the other cells of the immune system as well as to erythrocytes and platelets
Although the lymphocytes are responsible for adaptive immunity, all the other leukocytes play supporting roles in this specific response or are part of innate immunity.
- Monocytes give rise to the macrophages, and these along with the neutrophils are phagocytic cells.
- Eosinophils are important in the elimination of helminths (flatworms), either through the secretion of digestive enzymes through perforin pores inserted in the plasma membrane of the helminths or occasionally by phagocytosis. They also play a role in exacerbating chronic inflammatory diseases such as asthma or inflammatory bowel disease.
- Basophils and mast cells are not phagocytic but rather secrete inflammatory mediators such as histamine and prostaglandin in response to the binding of complement proteins during the elimination of pathogens. These cells, and mast cells in particular, are also activated during an allergic response, and the inflammatory mediators they release cause the symptoms of allergy.
- Dendritic cells are important in the activation of T cells, as will be described further. Dendritic cells also form a link between innate and adaptive immunity. Dendritic cells have a variety of TLRs that recognize pathogens and stimulate the secretion of cytokines and the inflammatory response. Thus, they can present antigens and recognize pathogen patterns via innate receptors as well.
In Adaptive Immunity, B lymphocytes and T lymphocytes Detect Foreign Molecules and Clear Them from the Body
A foreign molecule that triggers an adaptive immune response is called an antigen (“antibody generator”). Antigens are macromolecules; most are large proteins (including glycoproteins and lipoproteins) or polysaccharides (including lipopolysaccharides).
Some nucleic acids can also act as antigens, as can various large, artificially synthesized molecules.
Some antigens enter the body from the environment.
For example,
- Antigens on pathogens introduced beneath the skin,
- Antigens in vaccinations and inhaled or ingested macromolecules, such as toxins.
Other antigens are produced within the body
For example; Proteins encoded by viruses that have infected cells and altered proteins produced by mutated genes, such as those in cancer cells.
Two types of lymphocytes,
-
B cells
B cells differentiate from stem cells in the bone marrow. Differentiated B cells are released into the blood and carried to capillary beds serving the tissues and organs of the lymphatic system.
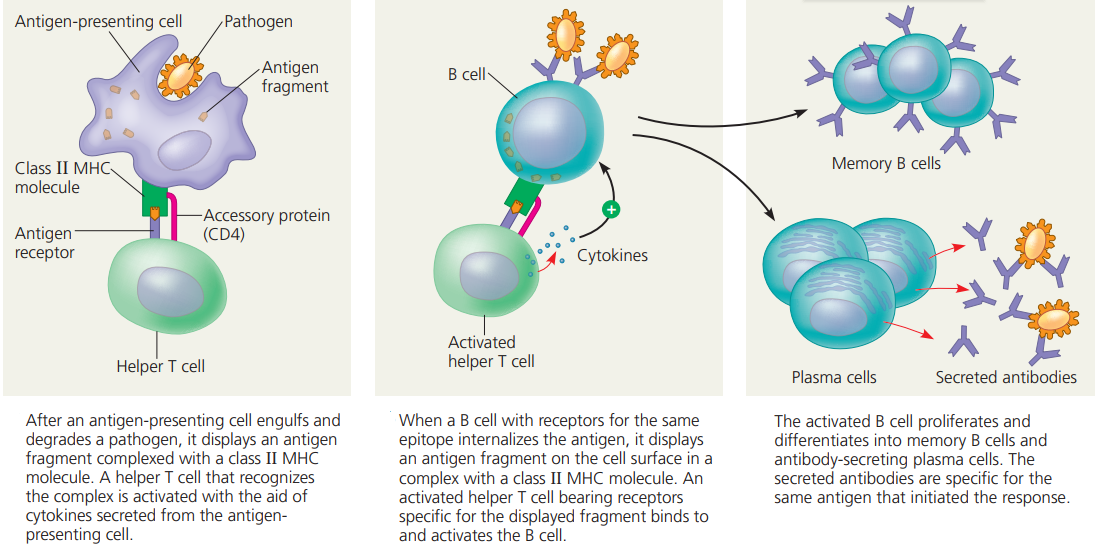
-
T cells
T cells originate from stem cells in the bone marrow. In immature form, they are released into the blood and carried to the thymus, where they mature. Two types of T cells
- helper T cells and
- cytotoxic T cells
The T-cells and B-cells are recognized antigens in the body and are involved in adaptive immunity.
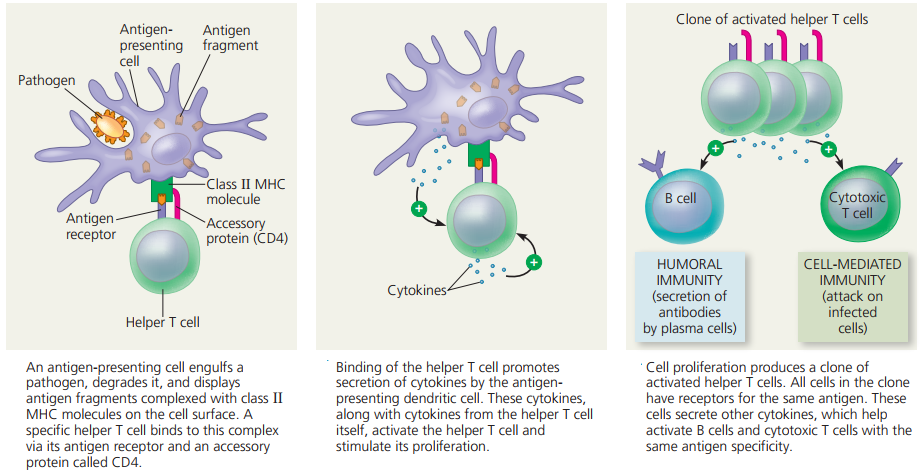
Adaptive immune responses
There are two types of adaptive immune responses:
Antibody-mediated immunity.
- In antibody-mediated immunity (also called humoral immunity).
- B-cell derivatives called plasma cells secrete antibodies, highly specific protein molecules that circulate in the blood and lymph recognizing and binding to antigens and clearing them from the body.
Cell-mediated immunity.
- In cell-mediated immunity, a particular type of T cell becomes activated and, with other cells of the immune system, attacks infected cells directly and kills them.
- The body’s reaction to an infection is not an either/or choice between antibody-mediated immunity and cell-mediated immunity.
- Rather, both responses typically occur, and, depending on the infection, the relative strength of the two responses varies.
- Complicated chemical signalling controls how the two responses develop.
The steps of the adaptive immune response are similar for antibody-mediated immunity and cell-mediated immunity:
-
Antigen encounter and recognition:
Lymphocytes encounter and recognize an antigen.
-
Lymphocyte activation:
The lymphocytes are activated by binding to the antigen and proliferate by cell division to produce many clones of identical cells.
-
Antigen clearance:
The many clones of activated lymphocytes clear the antigen from the body.
-
Development of immunological memory:
Some activated lymphocytes differentiate into memory cells that circulate in the blood and lymph, ready to initiate a rapid immune response upon later exposure to the same antigen.
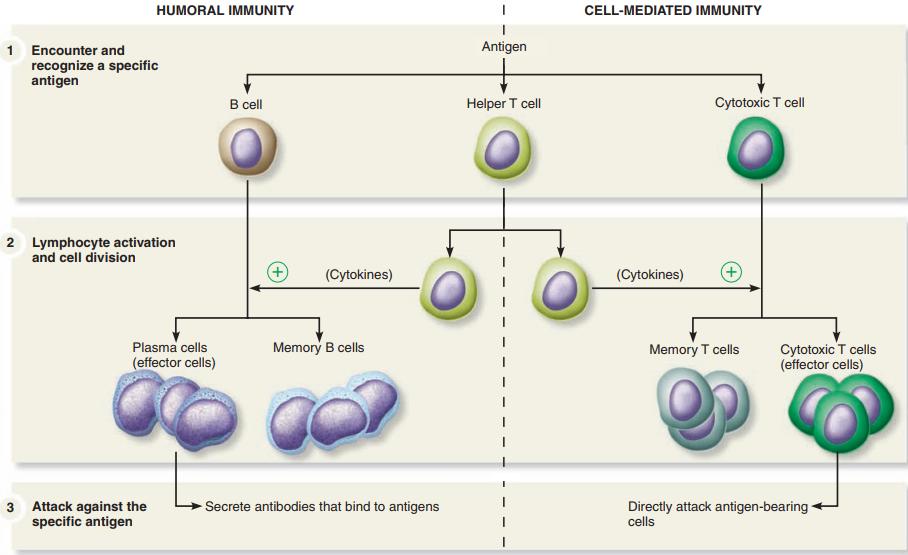
Antibody-Mediated Immunity.
- Antibody-mediated immunity begins as soon as an antigen is encountered and recognized in the body.
- Antigens are recognized by T cells that are activated as a result of the interaction and differentiate and proliferate, producing cells that activate B cells.
- Activated B cells differentiate into antibody-secreting cells.
- The antibodies are specific to the antigens that elicited the antibody-mediated immunity and they clear those antigens from the body.
ANTIGEN ENCOUNTER AND RECOGNITION BY LYMPHOCYTES
Antigens are encountered by B cells and T cells in the lymphatic system.
Each B cell and each T cell is specific for a particular antigen, meaning that the cell can bind to only one particular molecular structure.
The binding is specific because the plasma membrane of each B cell and T cell is studded with thousands of identical receptors for the antigen,
- the B-cell receptors on B-cells and
- the T-cell receptors on T-cells
B-cell receptors and T-cell receptors are encoded by different genes and thus have different structures.
The B-cell receptor on a B cell corresponds to the antibody secreted by that cell when it is activated and differentiates into a plasma cell.
Like its corresponding antibody, a B-cell receptor is a protein consisting of four polypeptide chains.
At one end, the protein has two identical antigen-binding sites, the regions that bind to a specific antigen.
At the opposite end of the antigen-binding sites are transmembrane domains, which are embedded in the plasma membrane.
T-cell receptors consist of a protein made up of two different polypeptides.
T-cell receptors also have an antigen-binding site at one end and transmembrane domains at the other end.
The binding between antigen and receptor is an interaction between two molecules that fit together like an enzyme and its substrate.
A given B-cell receptor or T-cell receptor does not bind to the whole antigen molecule but to small regions of it called epitopes or antigenic determinants.
Therefore, several different B cells and T cells, each with different receptors, may bind to the population of a particular antigen encountered in the lymphatic system.
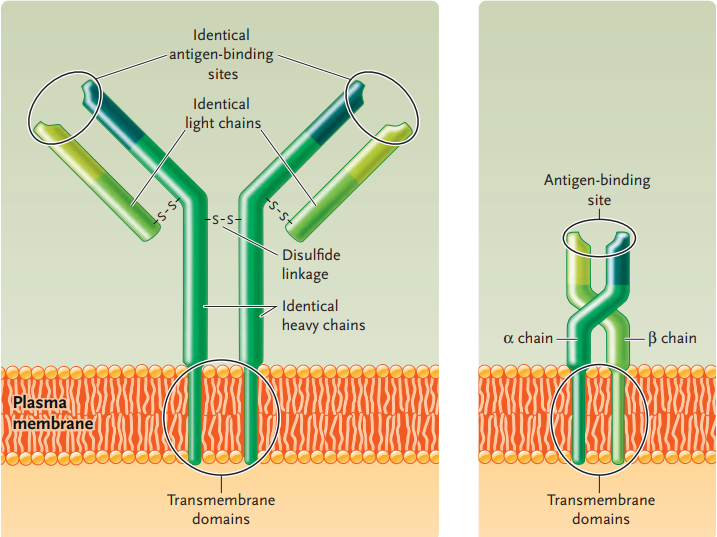
ANTIBODIES
They are the core molecules of antibody-mediated immunity.
Antibodies are large, complex Y-shaped molecules that belong to a class of proteins known as immunoglobulins (immunoglobulin [Ig]).
Each antibody molecule consists of four polypeptide chains:
- Two identical light chains
- And two identical heavy chains about twice or more the size of the light chain
Each polypeptide chain of an antibody molecule has
- a constant (C) region
- and a variable (V) region.
The constant region of each antibody type has the same amino acid sequence for that part of the heavy chain, and likewise for that part of the light chain.
The variable region of both the heavy and light chains, by contrast, has a different amino acid sequence for each antibody molecule in a population.
The variable regions are the top halves of the polypeptides in the arms of the Y-shaped molecule; the three-dimensional folding of the variable regions of each arm creates the antigen-binding site.
The antigen-binding site is the same for the two arms of an antibody molecule because its two heavy chains are identical, as are its two light chains, as mentioned earlier.
However, the antigen-binding sites are different from antibody molecule to antibody molecule because of the amino acid differences in the variable regions of the two chain types.
Different constant regions of the heavy chains in the tail part of the Y-shaped structure determine the antibody class.
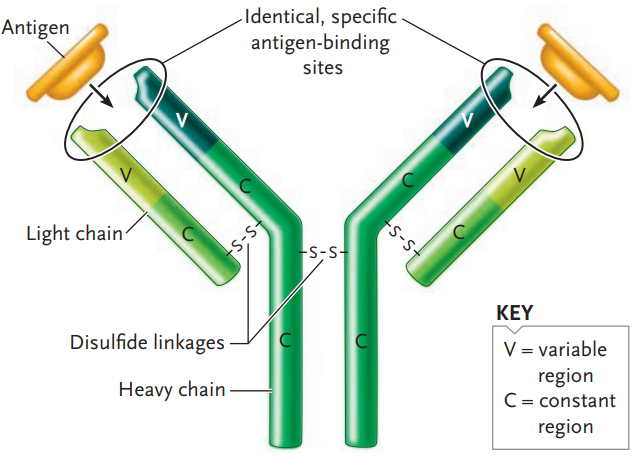
Humans have five different classes of antibodies
- IgM, IgG, IgA, IgE, and IgD.
The B-cell receptors on B cells are IgM molecules, and IgM is the first type of antibody secreted in an antibody-mediated immune response.
- IgG is the most abundant antibody circulating in the blood and lymphatic system. IgG is produced in large amounts when the body is exposed a second time to the same antigen. It also activates the complement system when it binds an antigen.
- IgA is found mainly in secretions at particular locations in the body where the antibodies it secretes bind to surface groups on pathogens and block their attachment to body surfaces.
- IgE is secreted by plasma cells of the skin and tissues lining the gastrointestinal tract and respiratory tract. IgE binds to basophils and mast cells, triggering the release of histamine, which causes an inflammatory response
- IgD also serves as a B-cell receptor.
THE GENERATION OF ANTIBODY DIVERSITY
The human genome has,19,900 protein-coding genes, far fewer than necessary to encode 100 million different antibodies if two genes encode one antibody, one gene for the heavy chain, and one for the light chain.
Instead, antibody diversity is generated during B-cell differentiation by a process involving three rearrangements of DNA segments that encode parts of the light and heavy chains.
The process that produces light-chain genes for an IgM antibody, the B-cell receptor; the production of IgM heavy-chain genes, and the light-chain and heavy-chain genes for the other classes of antibodies, is similar.
The genes for the two different subunits of the T-cell receptor undergo similar rearrangements to produce the great diversity in antigen-binding capability of those receptors.
Light-chain genes have one C segment, but heavy-chain genes have five types of C segments, each of which encodes one of the constant regions of IgM, IgD, IgG, IgE, and IgA.
In the assembly of functional heavy-chain genes, the inclusion of one of the five C segment types therefore specifies the class of anti- body that will be made by the cell.
Active Versus Passive Immunity
Active immunity
- It occurs when an individual produces a supply of antibodies.
- For example, when you catch measles, you recover because your body produces the antibodies that combine with the measles-causing viruses and bring about their destruction.
- Another way to become actively immune is to undergo immunization.
- Immunization involves the use of vaccines to bring about clonal expansion, not only of B cells but also of T cells.
- Traditionally, vaccines are the pathogens themselves, or their products, that have been treated so they are no longer virulent (able to cause disease).
- Vaccines against smallpox, polio, and tetanus have been successfully used worldwide.
- Today, it is possible to genetically engineer bacteria to mass-produce a protein from pathogens, and this protein can be used as a vaccine.
- This method was used to produce a vaccine against hepatitis B, a viral disease, and is being used to prepare a potential vaccine against malaria.
- After a vaccine is given, it is possible to determine the antibody titer (the amount of antibody present in a sample of plasma).
- After the first exposure to a vaccine, a primary response occurs.
- For several days, no antibodies are present; then the titer rises slowly, followed by first a plateau and then a gradual decline as the antibodies bind to the antigen or simply break down.
- After a second exposure, the titer rises rapidly to a plateau level much greater than before.
- The second exposure is called a “booster” because it boosts the antibody titer to a high level.
- The high antibody titer is expected to prevent disease symptoms when the individual is exposed to the antigen.
- Even years later, if the antigen enters the body, memory B cells quickly give rise to more plasma cells capable of producing the correct type of antibody.
Passive immunity
- Passive immunity occurs when an individual is given prepared antibodies (immunoglobulins) to combat a disease.
- Since these antibodies are not produced by the individual’s plasma cells, passive immunity is short-lived.
- For example, newborn infants are passively immune to some diseases because antibodies have crossed the placenta from the mother’s blood.
- These antibodies soon disappear, however, so that within a few months, infants become more susceptible to infections.
- Breastfeeding prolongs the natural passive immunity an infant receives from its mother because antibodies are present in the mother’s milk.
- Even though passive immunity does not last, it is sometimes used to prevent illness in a patient who has been unexpectedly exposed to an infectious disease.
- Artificial passive immunity is used in the emergency treatment of rabies, measles, tetanus, diphtheria, botulism, hepatitis A, and snakebites.
- Usually, the patient receives a gamma globulin injection (a serum that contains antibodies), extracted from a large, diverse adult population.
Join Enlighten Knowledge WhatsApp platform.
Join Enlighten Knowledge Telegram platform.