Why the first breath taken by a new born baby is so much harder than any subsequent breaths.
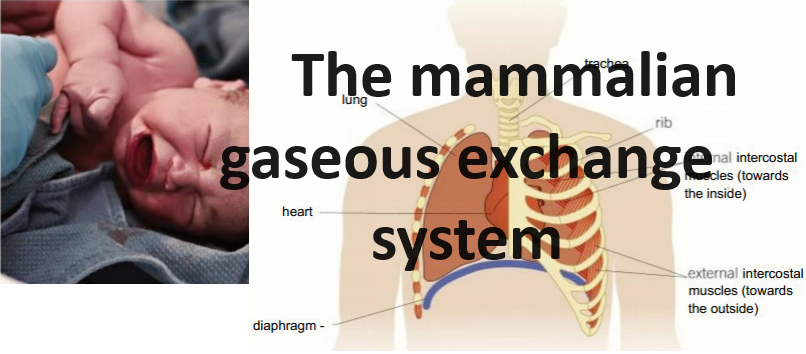
Why the first breath taken by a new born baby is so much harder than any subsequent breaths.
Content:
Introduction
The human gaseous exchange system
Key structures the human gaseous exchange system.
- Nasal cavity
- Trachea
- Bronchus
- Bronchioles
- Alveoli
The main adaptations of the alveoli for effective gaseous exchange.
Ventilating the lungs
- Inspiration
- Expiration
Why the first breath taken by a new born baby is so much harder than any subsequent breaths.
General characteristics of specialised exchange surfaces
Animals that live on the land face a continual conflict between the need for gaseous exchange and the need for water.
Gaseous exchange surfaces are moist, so oxygen dissolves in the waler before diffusing into the body tissues.
As a result, the conditions needed to take in oxygen successfully are also ideal for the evaporation of water.
Mammals have evolved complex systems that allow them to exchange gases efficiently but minimise the amount of water lost from the body.
The human gaseous exchange system
Mammals are relatively big; they have a small Surface Area to Volume (SA: V) ratio and a very large volume of cells.
They also have a high metabolic rate because they are active and maintain their body temperature independent of the environment.
As a result, they need lots of oxygen for cellular respiration and they produce carbon dioxide, which needs to be removed.
This exchange of gases takes place in the lungs.
Key structures the human gaseous exchange system.
Nasal cavity
The nasal cavity has a number of important features:
- a large surface area with a good blood supply, which warms the air to body temperature
- a hairy lining, which secretes mucus to trap dust and bacteria, protecting delicate lung tissue from irritation and infection
- moist surfaces, which increase the humidity of the incoming air, reducing evaporation from the exchange surfaces.
After passing through the nasal cavity, the air entering the lungs is a similar temperature and humidity to the air already there.
Trachea
The trachea is the main airway carrying clean, warm, moist air from the nose down into the chest.
It is a wide tube supported by incomplete rings of strong, flexible cartilage, which stop the trachea from collapsing.
The rings are incomplete so that food can move easily down the oesophagus behind the trachea.
The trachea and its branches are lined with a ciliated epithelium, with goblet cells between and below the epithelial cells.
Goblet cells secrete mucus onto the lining of the trachea, to trap dust and microorganisms that have escaped the nasal lining.
The cilia beat and move the mucus, along with any trapped dirt and microorganisms, away from the lungs.
Most of it goes into the throat and is swallowed and digested. One of the effects of cigarette smoke is that it stops these cilia beating.
Bronchus
In the chest cavity the trachea divides to form the left bronchus (plural bronchi), leading to the left lung, and the right bronchus leading to the right lung.
They are similar in structure to the trachea, with the same supporting rings of cartilage, but they are smaller.
Bronchioles
In the lungs the bronchi divide to form many small bronchioles.
The smaller bronchioles (diameter 1 mm or less) have no cartilage rings.
The walls of the bronchioles contain smooth muscle.
When the smooth muscle contracts, the bronchioles constrict (close up).
When it relaxes, the bronchioles dilate (open up).
This changes the amount of air reaching the lungs.
Bronchioles are lined with a thin layer of flattened epithelium, making some gaseous exchange possible.
Alveoli
The alveoli (singular alveolus) are tiny air sacs, which arc the main gas exchange surfaces of the body.
Alveoli are unique to mammalian lungs.
Each alveolus has a diameter of around 200-300 pm and consists of a layer of thin, flattened epithelial cells, along with some collagen and elastic fibres (composed of elastin).
These elastic tissues allow the alveoli to stretch as air is drawn in. When they return to their resting size, they help squeeze the air out.
This is known as the elastic recoil of the lungs.
The main adaptations of the alveoli for effective gaseous exchange include:
-
Large surface area
There are 300-500 million alveoli per adult lung.
The alveolar surface area for gaseous exchange in the two lungs combined is around 50-75 m2.
If the lungs were simple, balloon-like structures, the surface area would not be big enough for the amount of oxygen needed to diffuse into the body.
This demonstrates again the importance of the SA:V ratio.
-
Thin layers
Both the alveoli and the capillaries that surround them have walls that are only a single epithelial cell thick, so the diffusion distances between the air in the alveolus and the blood in the capillaries are very short.
-
Good blood supply
The millions of alveoli in each lung are supplied by a network of around 280 million capillaries.
The constant flow of blood through these capillaries brings carbon dioxide and carries off oxygen, maintaining a steep concentration gradient for both carbon dioxide and oxygen between the air in the alveoli and the blood in the capillaries.
-
Good ventilation
Breathing moves air in and out of the alveoli, helping maintain steep diffusion gradients lor oxygen and carbon dioxide between the blood and the air in the lungs.
The inner surface of the alveoli is covered in a thin layer of a solution of water, salts and lung surfactant.
It is this surfactant that makes it possible for the alveoli to remain inflated.
Oxygen dissolves in the water before diffusing into the blood, but water can also evaporate into the air in the alveoli.
Several of the adaptations of the human gas exchange system are to reduce this loss of water.
Ventilating the lungs
Air is moved in and out of the lungs as a result of pressure changes in the thorax (chest cavity) brought about by the breathing movements.
This movement of air is called ventilation.
The rib cage provides a semi-rigid case within which pressure can be lowered with respect to the air outside it.
The diaphragm is a broad, domed sheet of muscle, which forms the floor of the thorax.
The external intercostal muscles and the internal intercostal muscles are found between the ribs.
The thorax is lined by the pleural membranes, which surround the lungs.
The space between them, the pleural cavity, is usually filled with a thin layer of lubricating fluid so the membranes slide easily over each other as you breathe.
Inspiration (taking air in or inhalation)
The dome-shaped diaphragm contracts, flattening, and lowering.
The external intercostal muscles contract, moving the ribs upwards and outwards.
The volume of the thorax increases so the pressure in the thorax is reduced.
It is now lower than the pressure of the atmospheric air, so air is drawn through the nasal passages, trachea, bronchi, and bronchioles into the lungs.
This equalises the pressures inside and outside the chest.
Expiration
Normal expiration (breathing out or exhalation) is a passive process.
The muscles of the diaphragm relax so it moves up into its resting domed shape.
The external intercostal muscles relax so the ribs move down and inwards under gravity.
The elastic fibres in the alveoli of the lungs return to their normal length.
The effect of all these changes is to decrease the volume of the thorax.
Now the pressure inside the thorax is greater than the pressure of the atmospheric air, so air moves out of the lungs until the pressure inside and out is equal again.
You can exhale forcibly using energy.
The internal intercostal muscles contract, pulling the ribs down hard and fast, and the abdominal muscles contract forcing the diaphragm up to increase the pressure in the lungs rapidly.
Why the first breath taken by a new born baby is so much harder than any subsequent breaths
The first breath a newborn baby takes needs a force 15-20 times greater than any normal inhalation to inflate the lungs.
The lungs are enormously stretched as the air flows in, and the elastic tissue never returns to its original length.
This intake of breath is only possible because of special chemicals called lung surfactants containing phospholipids and both hydrophilic and hydrophobic proteins.
The surfactant stops the alveoli collapsing and sticking together as the baby exhales.
Without it, the second breath would be as difficult as the first, and continued breathing impossible.
Babies born at full term have alveoli coated in lung surfactant all ready for breathing.
However, the cells of the alveoli do not produce enough surfactant for the lungs to work properly until around the 30th week of pregnancy.
This is one reason why premature babies can struggle to breathe and may die.
In recent years artificial lung surfactants have been produced.
A tiny amount sprayed into the lungs of a premature baby coats the alveoli just like the natural surfactant, making breathing easier, helping to prevent lung damage and enabling many more babies to survive.
General characteristics of specialised exchange surfaces
Large, multicellular organisms have evolved specialised systems for the exchange of the substances they need and the substances they must remove.
All effective exchange surfaces have certain features in common. You will be looking at many of them in detail in this chapter.
Here is a summary of the characteristic features of effective exchange surfaces, along with some examples:
- Increased surface area
Provides the area needed for exchange and overcomes the limitations of the SA: V ratio of larger organisms.
Examples include root hair cells in plants and the villi in the small intestine of mammals.
- Thin layers
These mean the distances that substances have to diffuse are short, making the process fast and efficient.
Examples include the alveoli in the lungs and the villi of the small intestine.
- Good blood supply
The steeper the concentration gradient, the faster diffusion takes place. Having a good blood supply ensures substances are constantly delivered to and removed from the exchange surface.
This maintains a steep concentration gradient for diffusion.
Example, the alveoli of the lungs, the gills of a fish and the villi of the small intestine.
- Ventilation to maintain diffusion gradient
For gases, a ventilation system also helps maintain concentration gradients and makes the process more efficient,
For example, the alveoli and the gills of a fish where ventilation means a flow of water carrying dissolved gases.