Genetic Engineering, Gene Technology and Ethics
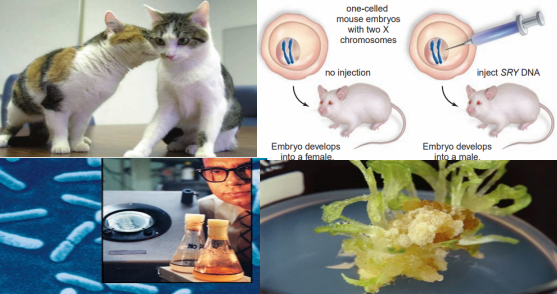
DNA sequencing and proteomics provide us with a detailed understanding of an organism’s genetic instructions.
Advances in these technologies and molecular biotechnology techniques mean it is now possible to manipulate an organism’s genome to achieve a desired outcome.
This manipulation of the genome is called genetic engineering.
The basic principles of genetic engineering involve isolating a gene for a desirable characteristic in one organism and placing it into another organism, using a suitable vector.
The two organisms between which the genes are transferred may be the same, similar, or very different species.
An organism that carries a gene from another organism is termed ‘transgenic’ and is often called a Genetically Modified Organism (GMO).
Isolating the desired gene
The first stage of successful genetic modification is to isolate the desirable gene.
The most common technique uses enzymes called restriction endonucleases to cut the required gene from the DNA of an organism.
Each type of endonuclease is restricted to breaking the DNA strands at specific base sequences within the molecule.
Some make a clean, blunt-ended cut in the DNA.
However, many restriction endonucleases cut the two DNA strands unevenly, leaving one of the strands of the DNA fragment a few bases longer than the other strand.
These regions with unpaired, exposed bases are called sticky ends.
The sticky ends make it much easier to insert the desired gene into the DNA of a different organism.
Another technique involves isolating the mRNA for the desired gene and using the enzyme reverse transcriptase to produce a single strand of complementary DNA.
The advantage of this technique is that it makes it easier to identify the desired gene, as a particular cell will make some very specific types of mRNA.
The formation of recombinant DNA
The DNA isolated by restriction endonucleases must be inserted into a vector that can carry it into the host cell.
Vectors
The most commonly used vectors in genetic engineering are bacterial plasmids – small circular molecules of DNA separate from the chromosomal DNA that can replicate independently.
Once a plasmid gets into a new host cell it can combine with the host DNA to form what is called recombinant DNA.
Plasmids are particularly effective in the formation of genetically engineered bacteria used, for example, to make human proteins.
The plasmids that are used as vectors are often chosen because they contain what is known as a marker gene.
For example, they may have been engineered to have a gene for antibiotic resistance.
This gene enables scientists to determine that the bacteria have taken up the plasmid, by growing the bacteria in media containing the antibiotic.
To insert a DNA fragment into a plasmid, first, it must be cut open.
The same restriction endonuclease used to isolate the DNA fragment is used to cut the plasmid.
This results in the plasmid having complementary sticky ends to the sticky ends of the DNA fragment.
Once the complementary bases of the two sticky ends are lined up.
The enzyme DNA ligase forms phosphodiester bonds between the sugar and the phosphate groups on the two strands of DNA, joining them together.
The plasmids used as vectors are usually given a second marker gene, which is used to show that the plasmid contains the recombinant gene.
This marker gene is often placed in the plasmid by genetic engineering methods.
The plasmid is then cut by a restriction enzyme within this marker gene to insert the desired gene.
If the DNA fragment is inserted successfully, the marker gene will not function.
In the early days of genetic engineering, these marker genes were often for antibiotic resistance.
There have, however, been many concerns about antibiotic resistance in genetically engineered organisms.
As a result, genes producing fluorescence or an enzyme that causes a colour change in a particular medium are now more widely used as marker genes.
If a bacterium does not fluoresce, or change the colour of the medium, then it has been engineered successfully and can be grown on.
Transferring the vector
The plasmid with the recombinant DNA must be transferred into the host cell in a process called transformation.
One method is to culture the bacterial cells and plasmids in a calcium-rich solution and increase the temperature.
This causes the bacterial membrane to become permeable and the plasmids can enter.
Another method of transformation is electroporation.
A small electrical current is applied to the bacteria.
This makes the membranes very porous and so the plasmids move into the cells.
Electroporation can also be used to get DNA fragments directly into eukaryotic cells.
The new DNA will pass through the cell membrane and the nuclear membrane to fuse with the nuclear DNA.
Although this technique is effective, the power of the electric current has to be carefully controlled or the membrane is permanently damaged or destroyed, which in turn destroys the whole cell.
It is less useful in whole organisms.
Electrofusion
Another way of producing genetically modified (GM) cells is electrofusion.
In electrofusion, tiny electric currents arc applied to the membranes of two different cells.
This fuses the cell and nuclear membranes of the two different cells together to form a hybrid or polyploid cell, containing DNA from both.
It is used successfully to produce GM plants.
Electrofusion is used differently in animal cells, which do not fuse as easily and effectively as plant cells.
Their membranes have different properties and polyploid animal cells especially polyploid mammalian cells do not usually survive in the body of a living organism.
However, electrofusion is important in the production of monoclonal antibodies.
A monoclonal antibody is produced by a combination of a cell producing one single type of antibody with a tumours cell, which means it divides rapidly in culture.
Monoclonal antibodies are now used to identify pathogens in both animals and plants, and in the treatment of a number of diseases including some forms of cancer.
Engineering in different organisms
The techniques of genetic engineering vary between different types of organisms but the principles are the same.
It is much easier to carry out genetic modification of prokaryotes than eukaryotes, and among the eukaryotes, plants are easier to work with than animals.
Engineering prokaryotes
Bacteria and other microorganisms have been genetically modified to produce many different substances that are useful to people.
These include hormones, for example insulin and human growth hormone, clotting factors for haemophiliacs, antibiotics, pure vaccines, and many of the enzymes used in industry.
Engineering plants
One method of genetically modifying plants uses Agrobacterium tumefaciens, a bacterium that causes tumours in healthy plants.
A desired gene for example, for pesticide production, herbicide resistance, drought resistance, or higher yield is placed in the plasmid of A. tumefaciens along with a marker gene, for example, for antibiotic resistance or fluorescence.
This is then carried directly into the plant cell DNA.
The transgenic plant cells form a callus, which is a mass of GM plant cells, each of which can be grown into a new transgenic plant.
Transgenic plant cells can also be produced by electrofusion.
The cells produced have chromosomes from both of the original cells and so are polyploid.
The cells that are fused may be from similar species, or very different ones.
The main stages in this process involve;
- removal of the plant cell wall by cellulases,
- electrofusion to form a new polyploid cell,
- the use of plant hormones to stimulate the growth of a new cell wall,
- followed by callus formation
- and the production of many cloned, transgenic plants.
Engineering animals
It is much harder to engineer the DNA of eukaryotic animals, especially mammals, than it is to modify bacteria or plants.
This is partly because animal cell membranes are less easy to manipulate than plant cell membranes.
However, it is an important technique both to enable animals to produce some medically important proteins and to try and cure human genetic diseases such as CF and Huntington’s disease.
Gene Technology and Ethics
The rapid development of gene technology in recent years has made many amazing scientific advances in the fields of genetic engineering and biotechnology possible but it has also raised a number of ethical issues.
All scientists have a responsibility to consider the moral and social values, or ethics, of their work.
These ethical considerations are important for many reasons including the protection of human rights, human health and safety, animal welfare, and the protection of the environment.
Ethical lapses in scientific research can not only cause harm but can also damage the public’s trust in scientists and their research.
This in turn can have significant implications on the advancement of knowledge and understanding.
Clear and open two-way communication between scientists and the public is vital for building trust and regulating research.
Genetic manipulation of microorganisms
Microorganisms, particularly bacteria, have been genetically modified or engineered to produce many different substances that are useful to people, including insulin and vaccines.
These substances can be produced in very large quantities in this way.
GM microorganisms are also used to store a living record of the DNA of another organism in DNA libraries.
DNA sequencing projects, such as the HGP enable scientists to build a collection of sequenced DNA fragments from one organism that is then stored (and propagated) in microorganisms (usually bacteria or yeast) through the process of genetic engineering.
These libraries serve as a source of DNA fragments for further genetic engineering applications or for further study of their function.
GM microorganisms are a widely used tool in research for developing novel medical treatments and industrial processes, as well as the development of gene technology itself.
Genetically engineered pathogens, however, are not widely used in these applications for the obvious health and safety of the researchers and the wider public.
In the few cases in that genetic modification of pathogens is carried out, this is usually for the purposes of medical and epidemiological research and is strictly regulated.
There is, of course, the concern that genetic engineering of pathogens could be used for the purposes of biological warfare.
Attempts to modify the genomes of pathogens to be more virulent, or to be resistant to all known treatments, raise serious ethical concerns and is a largely prohibited area of research except in specialized military research facilities.
Ethical concerns
Initially, some people were uncomfortable with inserting human genes into microorganisms but the pure human medicines, antibiotics, and enzymes produced are now seen as overwhelmingly beneficial.
They have been used safely for many years now.
As a result, there is relatively little ethical debate about the use of GM microorganisms except for the manipulation of pathogens in biological warfare.
Genetically Modified (GM) plants
Some people think the genetic modification of plants will help feed the ever-growing human population and overcome environmental issues including excess carbon dioxide production and pollution.
Others have major concerns about the process.
Insect resistance in GM soya beans
Soya beans are a major world crop around 250 million tonnes are produced each year and over half of the plants are from GM strains.
In one such modification, scientists have inserted a gene into soya beans so that they produce the Bt protein.
The Bt protein is toxic to many of the pest insects that attack the plant and is widely used as a pesticide by organic farmers.
One increasingly widely used strain of soya beans has been engineered to be resistant to a common weed killer and to contain Bt protein.
This means farmers can spray to get rid of weeds, making all the resources of light, water, and minerals available to the beans, and they do not need to use pesticides.
These plants could enable farmers to grow a much higher-yield crop of soya beans with less labour and less expense.
Patenting and technology transfer
One of the major concerns about GM crops is that people in less economically developed countries will be prevented from using them by patents and issues of technology transfer.
When someone discovers a new technique or invents something, they can apply for a legal patent, which means that no one else can use it without payment.
The people who most need the benefits of, for instance, drought- or flood-resistant crops, high yields, and added nutritional value may therefore be unable to afford the GM seed.
They also rely on harvesting seeds from one year to plant the next something that patenting may make impossible.
These concerns are based on evidence.
The company that developed the herbicide-resistant and pesticide-producing soya beans, have patented them so farmers can buy the beans from them and grow them to use or sell them for food or processing only in the year they are bought.
They cannot save the seed to grow again the next year and in 2013 this was upheld in the US Supreme Court.
Some organisations, however, such as the International Rice Research Institute (IRRI), work to develop engineered rice specifically to support farmers in less economically developed countries with whom they share the technological developments without patent constraints on seed harvesting.
For example, they have engineered flood-resistant ‘scuba’ rice, which gives 70-80% of maximum potential yield even if submerged for 2-3 weeks by flooding.
Genetically Modified animals
It is much harder to produce GM vertebrates, especially mammals, but scientists are researching the use of microinjections tiny particles of gold covered in ONA and modified viruses to carry new genes into animal DNA.
Such techniques are used with a number of goals in mind, including the transfer of disease resistance from one animal to another, or to modify physiology in farmed animals.
Some examples of GM animals:
- Swine fever-resistant pigs – in 2013 UK scientists successfully inserted a gene from wild African pigs into the early embryos of a European pig strain giving them immunity to otherwise fatal African swine fever.
- Faster-growing salmon – in the USA, GM Atlantic salmon have received genes from faster-growing Chinook salmon. The genes cause them to produce growth hormones all year round. They grow to full adult size in half the lime of conventional salmon, making them a very efficient food source.
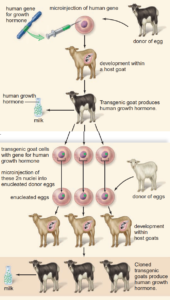
Pharming
One of the biggest uses of genetic engineering so far in animals is in the production of human medicines known as pharming.
There are two aspects to this field of gene technology:
- Creating animal models – the addition or removal of genes so that animals develop certain diseases, acting as models for the development of new therapies, for example, knockout mice have genes deleted so they are more likely to develop cancer.
- Creating human proteins – the introduction of a human gene coding for a medically required protein. Animals are sometimes used because bacteria cannot produce all of the complex proteins made by eukaryotic cells. The human gene can be introduced into the genetic material of a fertilised cow, sheep, or goat egg, along with a promoter sequence so the gene is expressed only in the mammary glands. The fertilised, transgenic female embryo is then returned to the mother. A transgenic animal is born and when it matures and gives birth, it produces milk. The milk will contain the desired human protein and can be harvested.
Ethical issues
There are many potential benefits to people and indeed to animal health of genetic engineering but the process also raises some ethical questions, which include:
- Should animals be genetically engineered to act as models of human disease?
- Is it right to put human genes into animals?
- Is it acceptable to put genes from another species into an animal without being certain it will not cause harm?
- Do genetically modifying animals reduce them to commodities?
- Is welfare compromised during the production of genetically engineered animals?
Gene therapy in humans
Some human diseases such as CF, haemophilia, and severe combined immunodeficiency (SCIDS) are the result of faulty (mutant) genes.
Scientists are looking at different ways of replacing the faulty allele with a healthy one.
They can remove the desired alleles from healthy cells or synthesise healthy alleles in the laboratory.
Somatic cell gene therapy
This involves replacing the mutant allele with a healthy allele in the affected somatic (body) cells.
The potential for helping people with a wide range of diseases is enormous.
Until recently there were few success stories as there were problems in;
- getting the healthy alleles into the affected cells,
- getting the engineered plasmids into the nucleus of the cells,
- and finally, difficulties in starting and maintaining expression of the healthy allele.
Viral vectors are often used.
In recent years, somatic cell gene therapy is beginning to show signs of fulfilling its potential.
Successful treatments have been reported for diseases including retinal disease (people have regained some vision), immune diseases, leukaemia, myelomas, and haemophilia.
The first gene therapy has recently (2012) been approved by the European Medicines Agency for lipoprotein lipase deficiency, which can cause severe pancreatitis.
However, somatic cell gene therapy is only a temporary solution for the treated individual.
The healthy allele will be passed on every time a cell divides by mitosis but somatic cells have a limited life and are replaced by stem cells, which will have the faulty allele.
In addition, a treated individual will still pass the faulty allele on to any children they have.
Germ-line cell gene therapy
The alternative to treating the somatic (body) cells of people already affected by a disease is to insert a healthy allele into the germ cells usually the eggs or into an embryo immediately after fertilisation (as part of in vitro fertilisation (IVF) treatment).
The individual would be born healthy with the normal allele in place and would pass it on to their own offspring.
This is called germ-line cell gene therapy.
Such therapy has been successfully done with animal embryos but is illegal for human embryos in most countries as a result of various ethical and medical concerns.
These concerns include the fact that the potential impact on an individual of an intervention on the germ cells is unknown.
Also, the human rights of the unborn individual could be said to be violated because it is, of course, done without consent and once done the process is irrevocable.
Another major ethical concern is that the technology might eventually be used to enable people to choose desirable or cosmetic characteristics of their offspring.